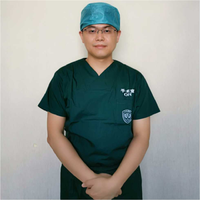
不只是瘦、弱:重视肌肉减少症(Sarcopenia)
不只是瘦、弱:重视肌肉减少症(Sarcopenia)
陶可(北京大学人民医院骨关节科)
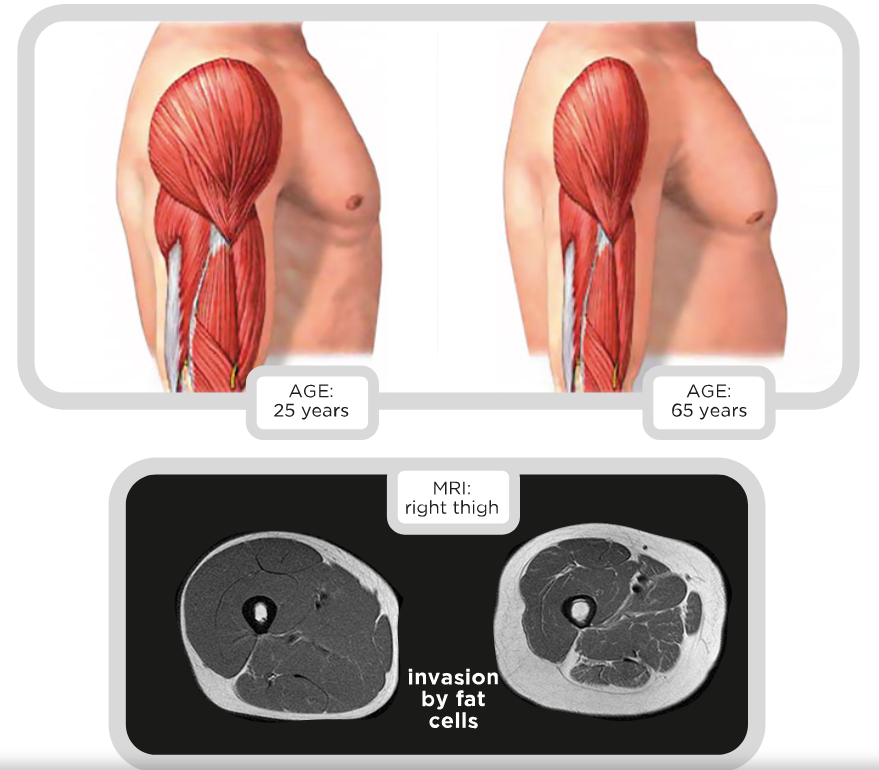
图1 25岁和65岁是的上臂肌肉大体结构、核磁共振MRI检查结果:可见25岁时肌肉较为丰富、发达;而65岁时,肌肉已经发生明显萎缩,质量变差,且MRI上可见较多量的脂肪组织细胞的浸润,替代了原来肌肉组织。从而,会导致人体肌肉力量衰退,活动量减少,平衡控制力降低,这可能也是跌倒已发生在老年人群的重要原因之一。
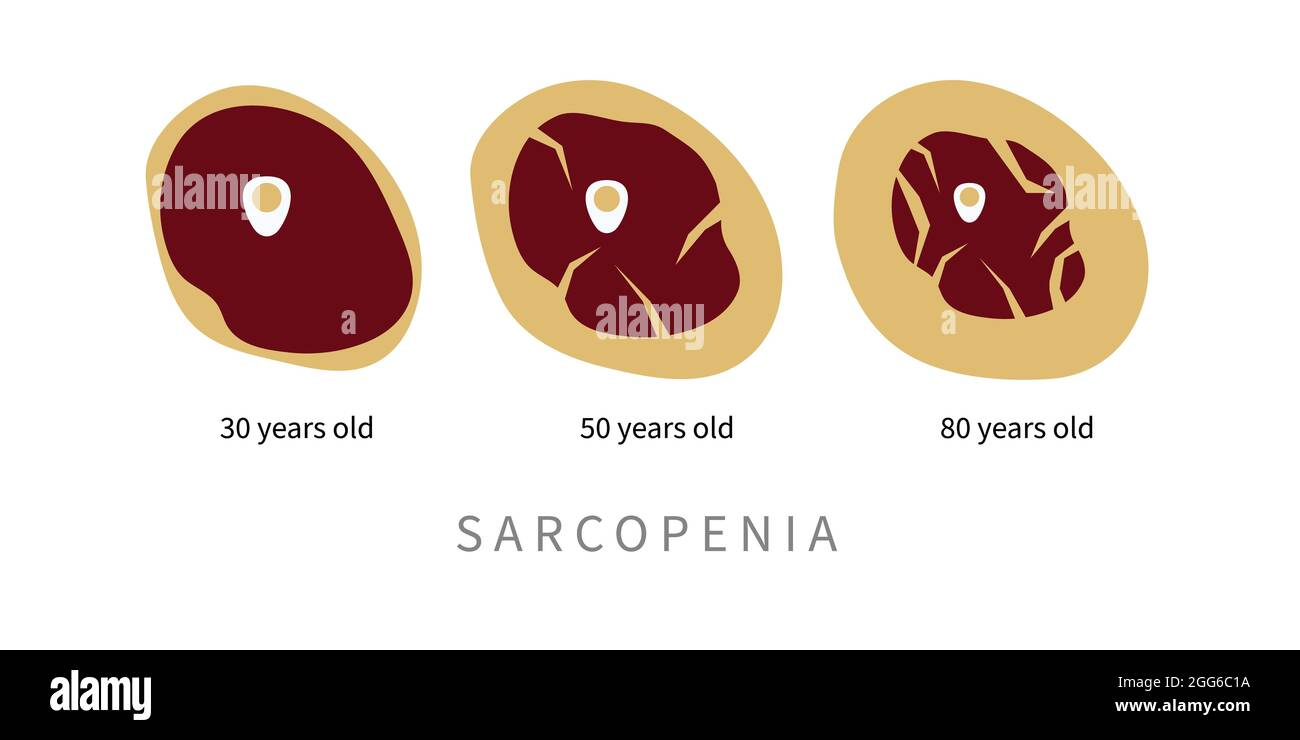
图2 30岁、50岁和80岁的肌肉(大腿前方股四头肌)比较:可见30岁时肌肉较为丰富、发达,围绕在骨骼周围;而50岁时,肌肉已经发生较为明显的萎缩,取而代之的是脂肪组织的浸润,同时可见骨骼组织也出现了部分萎缩;80岁的肌肉组织发生了非常显著的进一步萎缩,脂肪组织浸润较为明显,骨骼组织相应的也出现广泛萎缩,而肢体周经也出现一定的缩小。
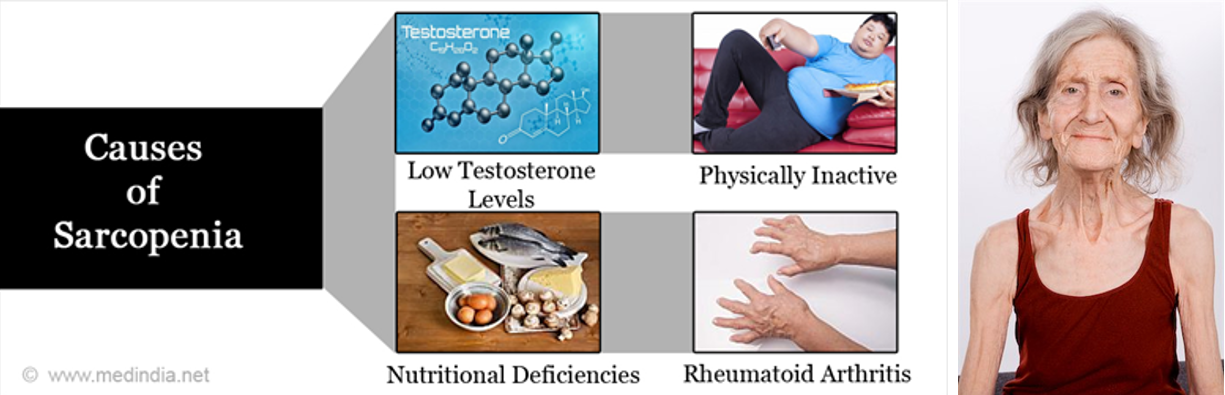
图3 肌肉萎缩症的主要病因包括:①low testosterone levels,低睾酮水平(雄性激素降低);②physically inactive,身体活动量低,缺乏有效体育锻炼;③nutritional deficiencies,营养缺乏或不良;④rheumatoid arthritis,类风湿关节炎等某些影响全身各系统器官与代谢的慢性疾病;⑤elder年龄增长衰老。
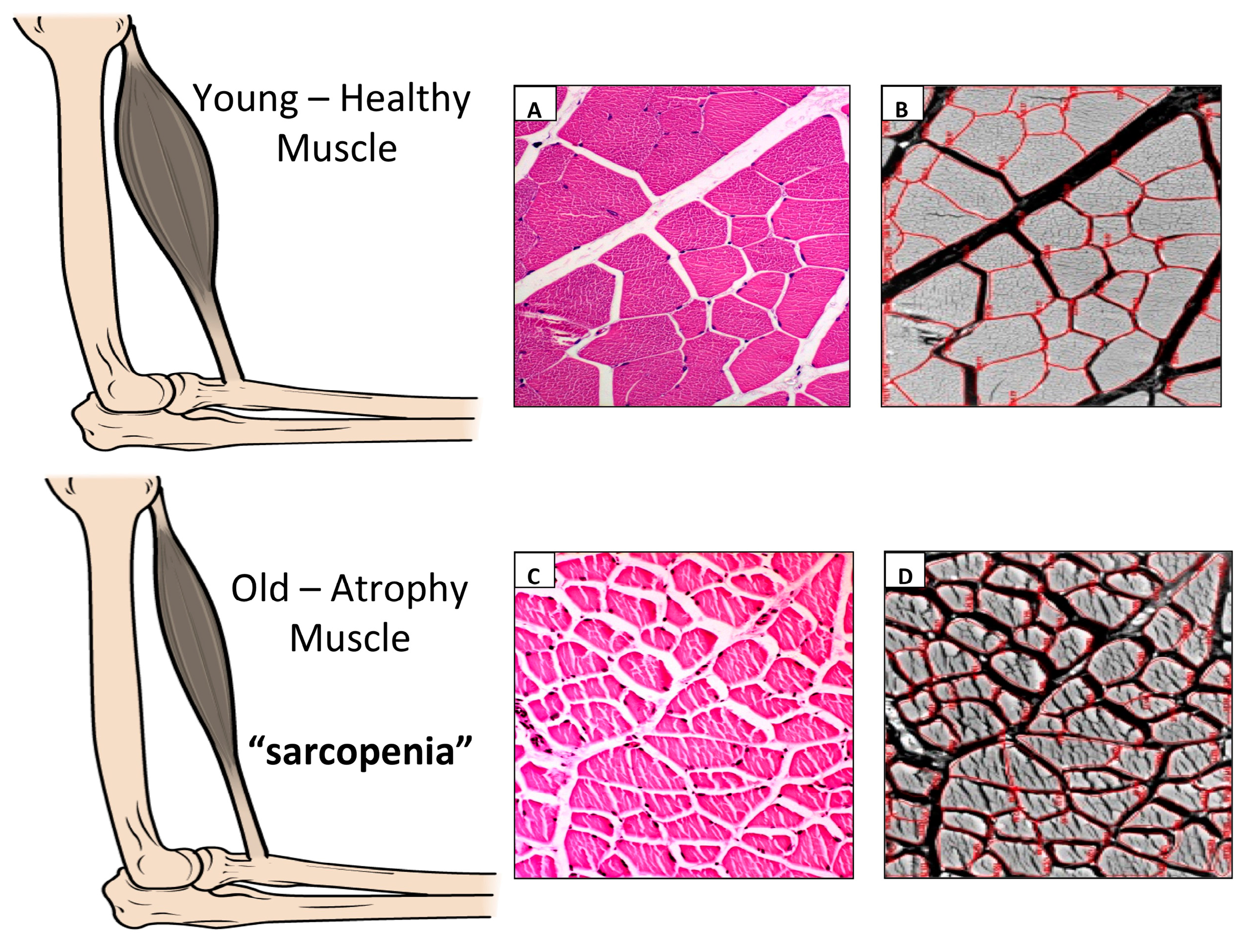
图4 (A)苏木精和曙红染色,以突出年轻、健康的Wistar大鼠肌肉组织中可能发生的结构改变。年轻健康大鼠的肌纤维组织结构未见任何损伤。(B)年轻健康Wistar大鼠肌肉纤维周 (μm)(平均值±标准差)的形态分析。在肌纤维周长(µm) (平均值±标准差)的形态分析中,年轻的健康大鼠显示出正常的肌肉营养。镜头倍率:20×。比例尺:50 µm;(C)苏木精和伊红染色以突出老年Wistar大鼠肌肉组织中可能发生的结构改变。老年大鼠肌纤维组织结构受损,表现为局灶性纤维化。(D)老年Wistar大鼠肌肉纤维周长(µm)(平均值±标准差)的形态学分析。在肌纤维周长(µm)(平均值±标准偏差)的形态分析中,老年大鼠表现出高度显着的肌纤维萎缩,并表现出显着的纤维尺寸异质性。使用图像采集软件(AxioVision Release 4.8.2-SP2 Software,Carl Zeiss Microscopy GmbH,Jena,Germany)考虑和计算肌肉纤维的周长。镜头倍率:20×。比例尺:50 µm。
肌肉减少症是一个重要的健康问题,涉及肌肉体积、质量和力量的逐渐下降。研究表明,它仅限于与年龄相关的肌肉质量和功能的改变。文献中的证据表明,低度慢性炎症易导致老年人肌肉减少症的进展。炎症标志物的测量可能表明老年人在多种疾病/健康状况下的功能限制。炎症是干预的潜在目标,以避免与衰老相关的肌肉无力。然而,锻炼仍然是预防肌肉减少症的关键策略。结果表明,有氧运动训练(aerobic exercise training, AET)或高强度间歇训练可以增强抗阻力训练RE对骨骼肌的影响。由于在某些情况下老年人不能或不愿意执行运动训练计划,因此正在开发替代性潜在治疗方法来对抗肌肉减少症。最近的临床证据表明,全身振动(WBV)和全身肌电刺激(WB-EMS)可以提高功能受损老年人的肌肉运动能力。后一种治疗方法对于那些有单调慢性病的患者也可以在家中进行。目前的科学贡献想向科学界强调适应老年人身体活动的积极作用,作为预防或治疗肌肉萎缩的一种可能的非药物治疗。即使这项科学贡献表明了运动对预防和治疗老年人肌肉减少症的有益作用,仍需要进一步研究以改善晚年的肌肉功能表现。
肌肉减少症定义
肌肉减少症是一种随着衰老和/或不动而发生的肌肉损失(肌肉萎缩)。它的特征是骨骼肌体积、质量和强度的退行性丧失。肌肉损失的速度取决于运动水平、合并症、营养和其他因素。肌肉损失与肌肉合成信号通路的变化有关。它不同于恶病质,在恶病质(肿瘤或感染等消耗性疾病)中肌肉通过细胞因子介导的降解,尽管这两种情况可能并存。肌肉减少症被认为是虚弱综合征的一个组成部分。[1]肌肉减少症可导致生活质量下降、跌倒、骨折和残疾。[2][3]肌肉减少症是改变与人口老龄化相关的身体成分的一个因素;某些肌肉区域预计会首先受到影响,特别是大腿前部和腹部肌肉。[2][4]在人口研究中,体重指数(BMI)被认为在老龄人口中下降,而生物电阻抗分析(BIA)显示体脂比例上升。[5]肌肉减少症sarcopenia一词来自希腊语σìρξ sarx,“肉体”和 πενία penia,“贫穷”。这是罗森伯格于1989年首次提出的,他写道:“可能没有任何一种与年龄相关的衰退特征能够更显着地影响步行、活动能力、热量卡路里摄入量以及整体营养摄入量和状态、独立性、呼吸等。”
体征和症状
肌肉减少症的标志是瘦肌肉量减少或肌肉萎缩。由于肥胖、脂肪量变化或水肿,身体成分的变化可能难以检测。体重、肢体或腰围的变化并不是肌肉质量变化的可靠指标。肌肉减少症还可能导致力量下降、功能下降和跌倒风险增加。肌肉减少症也可能没有任何症状,直到它很严重并且经常未被识别。[1]然而,研究表明,身体上部可能会发生肥大以补偿这种瘦肌肉质量的损失[2][6]因此,肌肉减少症发作的一个早期指标可能是大腿前部和腹部肌肉质量的显着损失。[2]
病因
肌肉减少症的病因有很多,很可能是多种相互作用因素的结果。对肌肉减少症病因的了解尚不完整,但体内激素水平变化、行动不便、与年龄相关的肌肉变化、营养和神经退行性变化都被认为是潜在的致病因素。[7]
肌肉减少症的程度由两个因素决定:肌肉质量的初始量和肌肉质量下降的速度。由于这些因素在人群中存在差异,进展速度和肌肉损失变得明显的阈值是可变的。[8]不动会显着增加肌肉流失的速度,即使在年轻人中也是如此。其他可能增加肌肉减少症进展速度的因素包括营养摄入减少、体力活动不足或慢性疾病。[1]此外,流行病学研究表明,早期环境(儿童及青少年时期肌肉储备量)影响可能对肌肉健康产生长期影响。例如,低出生体重是早期环境不佳的标志,与成年后肌肉质量和力量下降有关。[9][10][11]
病理生理学机制
已经提出了多种理论来解释肌肉减少症的肌肉变化机制,包括卫星细胞募集的变化、合成代谢信号的变化、蛋白质氧化、炎症和发育因素。肌肉减少症的病理变化包括肌肉组织质量下降,反映在肌肉纤维被脂肪替代、纤维化增加、肌肉代谢变化、氧化应激和神经肌肉接头退化[12]。肌纤维类型的分布在肌肉减少症肌肉中也会发生变化,导致II型肌纤维或“快肌”减少,而I型肌纤维或“慢肌”肌纤维几乎没有减少。去神经支配的II型纤维通常通过慢速I型纤维运动神经的神经再支配转化为I型纤维。[13]
在受伤或运动时未能激活卫星细胞也被认为是肌肉减少症的病理生理学原因之一。[12]此外,氧化的蛋白质会导致脂褐素和交联蛋白的积累,从而导致骨骼肌中非收缩性物质的积累,并导致肌肉减少症。[8]一个明显的保护因素是足够水平的蛋白质BNIP3,它可以防止细胞累积受损的线粒体。BNIP3的缺乏会导致肌肉发炎和萎缩。[14]
诊断
多个专家组提出了多种诊断标准,并且仍然是研究和争论的领域。尽管缺乏广泛接受的定义,肌肉减少症在2016年被指定为ICD-10代码(M62.84),将其识别为一种疾病状态。[15]
当患者的肌肉质量至少低于相关人群平均值的两个标准差并且行走速度较慢时,可以诊断为肌肉减少症。[16]欧洲老年人肌肉减少症工作组(EWGSOP)为肌肉减少症制定了广泛的临床定义,指定为存在低肌肉质量和低肌肉力量或低身体表现。[7]其他国际团体提出的标准包括步行速度、6分钟步行距离或握力等指标。[15]单独的握力也被提倡作为肌肉减少症的临床标志物,该标志物简单且具有成本效益并且具有良好的预测能力,尽管它没有提供全面的信息。[17]有一些肌肉减少症筛查工具可以评估患者报告的日常活动困难,例如步行、爬楼梯或从椅子上站起来,并已被证明可以预测肌肉减少症和不良功能结果。[18]
肌肉减少症治疗
锻炼
运动仍然是肌肉减少症的首选干预措施,但将研究结果转化为临床实践具有挑战性。运动的类型、持续时间和强度在研究之间是可变的,防止肌肉减少症的标准化运动处方。[19]缺乏锻炼是肌肉减少症的一个重要危险因素,锻炼可以显着减缓肌肉流失的速度。[20]运动可以成为一种有效的干预措施,因为老化的骨骼肌保留了合成蛋白质的能力以响应短期阻力运动。[21]老年人的渐进式阻力训练可以改善身体表现(步态速度)和肌肉力量。[22]
(锻炼方式,大家可参考:
下肢、膝关节疼痛、肿胀:说说膝关节核心肌群——股四头肌锻炼https://station.haodf.com/health/article?healthId=8618339876&articleId=9391847461)
药物
目前,尚无批准用于治疗肌肉减少症的药物。[23]睾酮或其他合成代谢类固醇也被研究用于治疗肌肉减少症,似乎对肌肉力量和质量有一些积极影响,但会引起一些副作用,并引起人们对男性前列腺癌和女性男性化的担忧。[24][25]此外,最近的研究表明,睾酮治疗可能会诱发不良心血管事件。[26][27][28] DHEA和人类生长激素已被证明在这种情况下几乎没有影响。生长激素增加肌肉蛋白质合成并增加肌肉质量,但在大多数研究中不会导致力量和功能的增加。[24]这一点,以及其效应胰岛素样生长因子1 (IGF-1)类似缺乏功效,可能是由于炎症和其他年龄变化导致衰老肌肉对IGF-1的局部抵抗。[24]
正在研究的其他可能用于治疗肌肉减少症的药物包括生长素释放肽、维生素D、血管紧张素转换酶抑制剂和二十碳五烯酸。[24][25]
营养
热量卡路里和蛋白质的摄入是肌肉蛋白质合成的重要刺激物。[29]老年人可能不像年轻人那样有效地利用蛋白质,并且可能需要更多的蛋白质来防止肌肉萎缩。[16]许多专家组建议将老年人的膳食蛋白质建议增加到每天1.0-1.2克/千克体重。[30][31]确保老年人有足够的营养有助于预防肌肉减少症和虚弱,因为这是一种简单、低成本的治疗方法,没有重大副作用。[32]
额外补充物
肌肉减少症的一个组成部分是老化骨骼肌丧失对合成代谢刺激(如氨基酸)作出反应的能力,尤其是在较低浓度时。然而,老化的肌肉保留了对较大剂量的蛋白质或氨基酸的合成代谢反应的能力。据报道,补充较大剂量的氨基酸,特别是亮氨酸可以抵消因衰老而导致的肌肉损失。[33]运动可以与补充氨基酸协同作用。[23]
β-羟基β-甲基丁酸(HMB)是亮氨酸的代谢物,可作为刺激蛋白质合成的信号分子。[16][23]据报道,它具有多个目标,包括刺激mTOR和降低蛋白酶体表达。临床试验始终支持使用它来防止瘦的老年人失去体重。[34][35][36]需要更多的研究来确定HMB对该年龄组肌肉力量和功能的精确影响。[35]
流行病学
肌肉减少症的患病率取决于每项流行病学研究中使用的定义。60-70岁人群的估计患病率为5-13%,80岁以上人群的患病率增加到11-50%。这相当于超过5000万人,预计在未来40年内将影响超过2亿人,因为老年人口不断增加。[7]
公共卫生影响
鉴于工业化人口的寿命延长和老年人口的增加,肌肉减少症正在成为一个主要的公共卫生问题。肌肉减少症是许多不良后果的预兆,包括增加残疾、跌倒和死亡率。易患肌肉减少症的人群不动或卧床休息会对功能结果产生巨大影响。在老年人中,这通常会导致生物储备减少和对压力源的脆弱性增加,称为“虚弱综合症”。瘦体重的减少还与感染风险增加、免疫力下降和伤口愈合不良有关。伴随肌肉萎缩的虚弱会导致跌倒、骨折、身体残疾、需要机构护理、生活质量下降、死亡率增加和医疗费用增加的风险增加。[16]这代表了重大的个人和社会负担,其公共卫生影响越来越受到认可。[7]
研究方向
有很多机会可以更好地了解肌肉减少症的原因和后果,并帮助指导临床护理。这包括阐明肌肉减少症的分子和细胞机制,按种族进一步细化参考人群,验证诊断标准和临床工具,以及跟踪住院率、发病率和死亡率。 还需要确定和研究潜在的治疗方法和干预时机。[37]
临床开发中的新药物疗法包括肌肉生长抑制素和选择性雄激素受体调节剂(SARM)。[38]非甾体选择性雄激素受体调节剂SARM特别令人感兴趣,因为它们在睾酮对肌肉的合成代谢作用之间表现出显着的选择性,但几乎没有雄激素作用的证据(例如男性前列腺刺激)。[38]
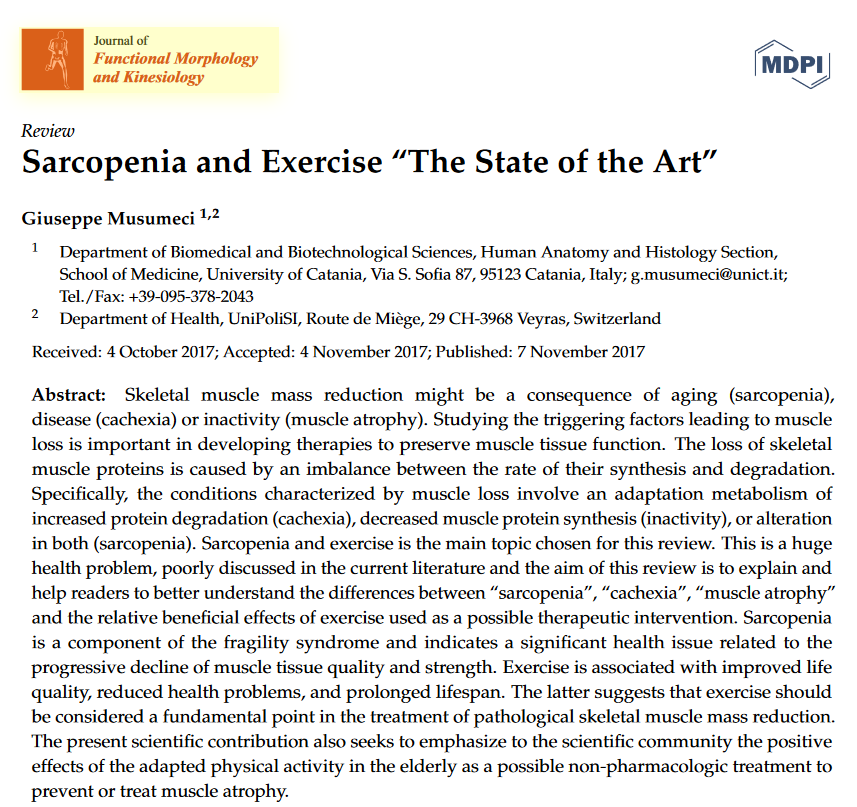
Sarcopenia
Sarcopenia is an important health problem involving a progressive decline of muscle mass, quality and strength. It has been shown that it is limited to the age-related alteration of muscle mass and function. Evidence in literature suggests that low-grade chronic inflammation predisposes to the progress of sarcopenia in the elderly. The measurement of inflammatory markers may be indicative of functional limitations in older people across several diseases/health conditions. Inflammation is a potential target for interventions to avoid muscular weakness associated with ageing. However, exercise continue to be the key strategy to prevent sarcopenia. It was shown that aerobic exercise training AET or high-intensity interval training may enhance the effects of resistance exercise (RE) on skeletal muscle. Since older adults are unable or unwilling to perform exercise training programs in some cases, alternative potential treatment approaches are being developed to counter the sarcopenia. Recent clinical evidence has shown that whole-body vibration (WBV) and whole-body electromyostimulation (WB-EMS) can improve muscle exercise capacity in functionally impaired older people. The latter kind of therapeutic treatment could also be carried out at home for those patients who have monotonous chronic problems. The present scientific contribution would like to emphasize to the scientific community the positive effects of the adapted physical activity in the elderly as a possible non-pharmacologic treatment to prevent or treat muscle atrophy. Even if this scientific contribution has suggested the beneficial effects of exercise for the prevention and treatment of sarcopenia in elderly people, further studies are required to improve muscle performance in later life.
Sarcopenia is a type of muscle loss (muscle atrophy) that occurs with aging and/or immobility. It is characterized by the degenerative loss of skeletal muscle mass, quality, and strength. The rate of muscle loss is dependent on exercise level, co-morbidities, nutrition and other factors. The muscle loss is related to changes in muscle synthesis signalling pathways. It is distinct from cachexia, in which muscle is degraded through cytokine-mediated degradation, although both conditions may co-exist. Sarcopenia is considered a component of frailty syndrome.[1] Sarcopenia can lead to reduced quality of life, falls, fracture, and disability.[2][3]
Sarcopenia is a factor in changing body composition associated with aging populations; and certain muscle regions are expected to be affected first, specifically the anterior thigh and abdominal muscles.[2][4] In population studies, body mass index (BMI) is seen to decrease in aging populations while bioelectrical impedance analysis (BIA) shows body fat proportion rising.[5]
The term sarcopenia is from Greek σάρξ sarx, “flesh“ and πενία penia, “poverty“. This was first proposed by Rosenberg in 1989, who wrote that “there may be no single feature of age-related decline that could more dramatically affect ambulation, mobility, calorie intake, and overall nutrient intake and status, independence, breathing, etc.“
Signs and symptoms
The hallmark sign of sarcopenia is loss of lean muscle mass, or muscle atrophy. The change in body composition may be difficult to detect due to obesity, changes in fat mass, or edema. Changes in weight, limb or waist circumference are not reliable indicators of muscle mass changes. Sarcopenia may also cause reduced strength, functional decline and increased risk of falling. Sarcopenia may also have no symptoms until it is severe and is often unrecognized.[1] Research has shown, however, that hypertrophy may occur in the upper parts of the body to compensate for this loss of lean muscle mass[2][6] Therefore, one early indicator of the onset of sarcopenia can be significant loss of muscle mass in the anterior thigh and abdominal muscles.[2]
Causes
There are many proposed causes of sarcopenia and it is likely the result of multiple interacting factors. Understanding of the causes of sarcopenia is incomplete, however changes in hormones, immobility, age-related muscle changes, nutrition and neurodegenerative changes have all been recognized as potential causative factors.[7]
The degree of sarcopenia is determined by two factors: initial amount of muscle mass and rate at which muscle mass declines. Due to variations in these factors across the population, the rate of progression and the threshold at which muscle loss becomes apparent is variable.[8] Immobility dramatically increases the rate of muscle loss, even in younger people. Other factors that can increase rate of progression of sarcopenia include decreased nutrient intake, low physical activity, or chronic disease.[1] Additionally, epidemiological research has indicated that early environmental influences may have long-term effects on muscle health. For example, low birth weight, a marker of a poor early environment, is associated with reduced muscle mass and strength in adult life.[9][10][11]
Pathophysiology
There are multiple theories proposed to explain the mechanisms of muscle changes of sarcopenia including changes in satellite cell recruitment, changes in anabolic signalling, protein oxidation, inflammation, and developmental factors. The pathologic changes of sarcopenia include a reduction in muscle tissue quality as reflected in the replacement of muscle fibers with fat, an increase in fibrosis, changes in muscle metabolism, oxidative stress, and degeneration of the neuromuscular junction.[12]
The distribution of muscle fibers types also changes in sarcopenic muscle causing a decrease in type II muscle fibers, or “fast twitch,“ with little to no decrease in type I muscle fibers, or “slow-twitch“ muscle fibers. Deinervated type II fibers are often converted to type I fibers by reinnervation by slow type I fiber motor nerves.[13]
The failure to activate satellite cells upon injury or exercise is also thought to contribute to the pathophysiology of sarcopenia.[12] Additionally, oxidized proteins can lead to a buildup of lipofuscin and cross-linked proteins causing an accumulation of non-contractile material in the skeletal muscle and contribute to sarcopenic muscle.[8]
An apparent protective factor is sufficient levels of the protein BNIP3, which prevents cells‘ buildup of damaged mitochondria. Deficiency of BNIP3 leads to muscle inflammation and atrophy.[14]
Diagnosis
Multiple diagnostic criteria have been proposed by various expert groups and continues to be an area of research and debate. Despite the lack of a widely accepted definition, sarcopenia was assigned an ICD-10 code (M62.84) in 2016, recognizing it as a disease state.[15]
Sarcopenia can be diagnosed when a patient has muscle mass that is at least two standard deviations below the relevant population mean and has a slow walking speed.[16] The European Working Group on Sarcopenia in Older People (EWGSOP) developed a broad clinical definition for sarcopenia, designated as the presence of low muscle mass and either low muscular strength or low physical performance.[7] Other international groups have proposed criteria that include metrics on walking speed, distance walked in 6 minutes, or grip strength.[15] Hand grip strength alone has also been advocated as a clinical marker of sarcopenia that is simple and cost effective and has good predictive power, although it does not provide comprehensive information.[17]
There are screening tools for sarcopenia that assess patient reported difficulty in doing daily activities such as walking, climbing stairs or standing from a chair and have been shown to predict sarcopenia and poor functional outcomes.[18]
Management
Exercise
Exercise remains the intervention of choice for sarcopenia, but translation of research findings into clinical practice is challenging. The type, duration and intensity of exercise are variable between studies, preventing a standardized exercise prescription for sarcopenia.[19] Lack of exercise is a significant risk factor for sarcopenia and exercise can dramatically slow the rate of muscle loss.[20] Exercise can be an effective intervention because aging skeletal muscle retains ability to synthesize proteins in response to short-term resistance exercise.[21] Progressive resistance training in older adults can improve physical performance (gait speed) and muscular strength.[22]
Medication
There are currently no approved medications for the treatment of sarcopenia.[23] Testosterone or other anabolic steroids have also been investigated for treatment of sarcopenia, and seem to have some positive effects on muscle strength and mass, but cause several side effects and raise concerns of prostate cancer in men and virilization in women.[24][25] Additionally, recent studies suggest testosterone treatments may induce adverse cardiovascular events.[26][27][28]
DHEA and human growth hormone have been shown to have little to no effect in this setting. Growth hormone increases muscle protein synthesis and increases muscle mass, but does not lead to gains in strength and function in most studies.[24] This, and the similar lack of efficacy of its effector insulin-like growth factor 1 (IGF-1), may be due to local resistance to IGF-1 in aging muscle, resulting from inflammation and other age changes.[24]
Other medications under investigation as possible treatments for sarcopenia include ghrelin, vitamin D, angiotensin converting enzyme inhibitors, and eicosapentaenoic acid.[24][25]
Nutrition
Intake of calories and protein are important stimuli for muscle protein synthesis.[29] Older adults may not utilize protein so efficiently as younger people and may require higher amounts to prevent muscle atrophy.[16] A number of expert groups have proposed an increase in dietary protein recommendations for older age groups to 1.0-1.2 g/kg body weight per day.[30][31] Ensuring adequate nutrition in older adults is of interest in the prevention of sarcopenia and frailty, since it is a simple, low-cost treatment approach without major side effects.[32]
Supplements
A component of sarcopenia is the loss of ability for aging skeletal muscle to respond to anabolic stimuli such as amino acids, especially at lower concentrations. However, aging muscle retains the ability of an anabolic response to protein or amino acids at larger doses. Supplementation with larger doses of amino acids, particularly leucine has been reported to counteract muscle loss with aging.[33] Exercise may work synergistically with amino acid supplementation.[23]
β-hydroxy β-methylbutyrate (HMB) is a metabolite of leucine that acts as a signalling molecule to stimulate protein synthesis.[16][23] It is reported to have multiple targets, including stimulating mTOR and decreasing proteasome expression. Its use to prevent the loss of lean body mass in older adults is consistently supported in clinical trials.[34][35][36] More research is needed to determine the precise effects of HMB on muscle strength and function in this age group.[35]
Epidemiology
The prevalence of sarcopenia depends on the definition used in each epidemiological study. Estimated prevalence in people between the ages of 60-70 is 5-13% and increases to 11-50% in people more than 80 years of age. This equates to >50 million people and is projected to affect >200 million in the next 40 years given the rising population of older adults.[7]
Public health impact
Sarcopenia is emerging as a major public health concern given the increased longevity of industrialized populations and growing geriatric population. Sarcopenia is a predictor of many adverse outcomes including increased disability, falls and mortality[citation needed]. Immobility or bed rest in populations predisposed to sarcopenia can cause dramatic impact on functional outcomes. In the elderly, this often leads to decreased biological reserve and increased vulnerability to stressors known as the “frailty syndrome“. Loss of lean body mass is also associated with increased risk of infection, decreased immunity, and poor wound healing. The weakness that accompanies muscle atrophy leads to higher risk of falls, fractures, physical disability, need for institutional care, reduced quality of life, increased mortality, and increased healthcare costs.[16] This represents a significant personal and societal burden and its public health impact is increasingly recognized.[7]
Research directions
There are significant opportunities to better understand the causes and consequences of sarcopenia and help guide clinical care. This includes elucidation of the molecular and cellular mechanisms of sarcopenia, further refinement of reference populations by ethnic groups, validation of diagnostic criteria and clinical tools, as well as tracking of incidence of hospitalization admissions, morbidity, and mortality. Identification and research on potential therapeutic approaches and timing of interventions is also needed.[37]
New pharmaceutical therapies in clinical development include myostatin and the selective androgen receptor modulators (SARMs).[38] Nonsteriodal SARMs are of particular interest, given they exhibit significant selectivity between the anabolic effects of testosterone on muscle, but with little to no evidence of androgenic effects (such as prostate stimulation in men).[38]
Figure 4. (A) Hematoxylin & Eosin staining in order to highlight possible structural alterations in muscle tissue from young, healthy Wistar Rats. Muscle fibers of young healthy rat did not show any damaged histological structure. The samples were examined with a Zeiss Axioplan light microscope (Carl Zeiss, Oberkochen, Germany) and a digital camera (AxioCam MRc5, Carl Zeiss) was used to take the pictures. Lens magnification: 20×. Scale bars: 50 µm; (B) Morphometric analysis of the perimeter (μm) (mean ± SD) of the muscle fibers from young healthy Wistar Rats. In the morphometric analysis of the perimeter (µm) (mean ± SD) of the muscle fibers, the young healthy rat shows normal muscle trophic. The perimeter of muscle fibers was considered and calculated using software for image acquisition (AxioVision Release 4.8.2-SP2 Software, Carl Zeiss Microscopy GmbH, Jena, Germany). Lens magnification: 20×. Scale bars: 50 µm; (C) Hematoxylin & Eosin staining in order to highlight possible structural alterations in muscle tissue from elderly Wistar Rats. Muscle fibers of elderly rat show damaged histological structure as focal perimisio fibrosis. The samples were examined with a Zeiss Axioplan light microscope (Carl Zeiss, Oberkochen, Germany) and a digital camera (AxioCam MRc5, Carl Zeiss) was used to take the pictures. Lens magnification: 20×. Scale bars: 50 µm; (D) Morphometric analysis of the perimeter (µm) (mean ± SD) of the muscle fibers from elderly Wistar Rats. In the morphometric analysis of the perimeter (µm) (mean ± SD) of the muscle fibers, the elderly rat shows a highly significant muscle fiber hypotrophy and exhibits remarkable fiber size heterogeneity. The perimeter of muscle fibers was considered and calculated using software for image acquisition (AxioVision Release 4.8.2-SP2 Software, Carl Zeiss Microscopy GmbH, Jena, Germany). Lens magnification: 20×. Scale bars: 50 µm.
From Wikipedia, the free encyclopedia
https://amino-ther.com/clinical-evidence/sarcopenia-and-frailty/
更多参考文献References
Peterson SJ, Mozer M (February 2017). “Differentiating Sarcopenia and Cachexia Among Patients With Cancer“. Nutrition in Clinical Practice. 32 (1): 30–39. doi:10.1177/0884533616680354. PMID 28124947. S2CID 206555460.
Ata AM, Kara M, Kaymak B, Ozcakar L. Sarcopenia Is Not “Love“: You Have to Look Where You Lost it!. Am J Phys Med Rehabil. 2020;99(10):e119-e120. doi:10.1097/PHM.0000000000001391.
Beaudart C, Zaaria M, Pasleau F, et al: Health outcomes of sarcopenia: a systematic reviewand meta-analysis.PLoS One2017;12:e0169548
Ata AM, Kara M, Kaymak B, et al: Regional and total muscle mass, muscle strength andphysical performance: the potential use of ultrasound imaging for sarcopenia.Arch GerontolGeriatr2019;83:55–60
Ranasinghe, Chathuranga; Gamage, Prasanna (2013). “Relationship between Body mass index (BMI) and body fat percentage, estimated by bioelectrical impedance, in a group of Sri Lankan adults: a cross sectional study“. BMC Public Health. 13: 797. doi:10.1186/1471-2458-13-797. PMC 3766672. PMID 24004464.
Özkal Ö, Kara M, Topuz S, et al: Assessment of core and lower limb muscles forstatic/dynamic balance in the older people: an ultrasonographic study.Age Ageing2019;48:881–7
Cruz-Jentoft AJ, Baeyens JP, Bauer JM, Boirie Y, Cederholm T, Landi F, et al. (July 2010). “Sarcopenia: European consensus on definition and diagnosis: Report of the European Working Group on Sarcopenia in Older People“. Age and Ageing. 39 (4): 412–23. doi:10.1093/ageing/afq034. PMC 2886201. PMID 20392703.
Marcell TJ (October 2003). “Sarcopenia: causes, consequences, and preventions“. The Journals of Gerontology. Series A, Biological Sciences and Medical Sciences (Review). 58 (10): M911-6. doi:10.1093/gerona/58.10.m911. PMID 14570858.
Sayer AA, Syddall HE, Gilbody HJ, Dennison EM, Cooper C (September 2004). “Does sarcopenia originate in early life? Findings from the Hertfordshire cohort study“. The Journals of Gerontology. Series A, Biological Sciences and Medical Sciences. 59 (9): M930-4. doi:10.1093/gerona/59.9.M930. PMID 15472158.
Gale CR, Martyn CN, Kellingray S, Eastell R, Cooper C (January 2001). “Intrauterine programming of adult body composition“. The Journal of Clinical Endocrinology and Metabolism. 86 (1): 267–72. doi:10.1210/jcem.86.1.7155. PMID 11232011.
Ylihärsilä H, Kajantie E, Osmond C, Forsén T, Barker DJ, Eriksson JG (September 2007). “Birth size, adult body composition and muscle strength in later life“. International Journal of Obesity. 31 (9): 1392–9. doi:10.1038/sj.ijo.0803612. PMID 17356523.
Ryall JG, Schertzer JD, Lynch GS (August 2008). “Cellular and molecular mechanisms underlying age-related skeletal muscle wasting and weakness“. Biogerontology (Review). 9 (4): 213–28. doi:10.1007/s10522-008-9131-0. PMID 18299960. S2CID 8576449.
Doherty TJ (October 2003). “Invited review: Aging and sarcopenia“. Journal of Applied Physiology (Review). 95 (4): 1717–27. doi:10.1152/japplphysiol.00347.2003. PMID 12970377.
Irazoki A; et al. (9 March 2022). “Coordination of mitochondrial and lysosomal homeostasis mitigates inflammation and muscle atrophy during aging“. Aging Cell. 21 (4): e13583. doi:10.1111/acel.13583. PMC 9009131. PMID 35263007.
Anker SD, Morley JE, von Haehling S (December 2016). “Welcome to the ICD-10 code for sarcopenia“. Journal of Cachexia, Sarcopenia and Muscle. 7 (5): 512–514. doi:10.1002/jcsm.12147. PMC 5114626. PMID 27891296.
Argilés JM, Campos N, Lopez-Pedrosa JM, Rueda R, Rodriguez-Mañas L (September 2016). “Skeletal Muscle Regulates Metabolism via Interorgan Crosstalk: Roles in Health and Disease“. Journal of the American Medical Directors Association. 17 (9): 789–96. doi:10.1016/j.jamda.2016.04.019. PMID 27324808.
Sayer AA (August 2010). “Sarcopenia“. BMJ. 341 (aug10 2): c4097. doi:10.1136/bmj.c4097. PMID 20699307. S2CID 220113690.
Malmstrom TK, Miller DK, Simonsick EM, Ferrucci L, Morley JE (March 2016). “SARC-F: a symptom score to predict persons with sarcopenia at risk for poor functional outcomes“. Journal of Cachexia, Sarcopenia and Muscle. 7 (1): 28–36. doi:10.1002/jcsm.12048. PMC 4799853. PMID 27066316.
Sayer AA (November 2014). “Sarcopenia the new geriatric giant: time to translate research findings into clinical practice“. Age and Ageing. 43 (6): 736–7. doi:10.1093/ageing/afu118. PMID 25227204.
Abate M, Di Iorio A, Di Renzo D, Paganelli R, Saggini R, Abate G (September 2007). “Frailty in the elderly: the physical dimension“. Europa Medicophysica. 43 (3): 407–15. PMID 17117147.
Yarasheski KE (October 2003). “Exercise, aging, and muscle protein metabolism“. The Journals of Gerontology. Series A, Biological Sciences and Medical Sciences (Review). 58 (10): M918-22. doi:10.1093/gerona/58.10.m918. PMID 14570859.
Liu CJ, Latham NK (July 2009). “Progressive resistance strength training for improving physical function in older adults“. The Cochrane Database of Systematic Reviews (3): CD002759. doi:10.1002/14651858.cd002759.pub2. PMC 4324332. PMID 19588334.
Phillips SM (July 2015). “Nutritional supplements in support of resistance exercise to counter age-related sarcopenia“. Advances in Nutrition. 6 (4): 452–60. doi:10.3945/an.115.008367. PMC 4496741. PMID 26178029.
Sakuma K, Yamaguchi A (28 May 2012). “Sarcopenia and age-related endocrine function“. International Journal of Endocrinology. 2012: 127362. doi:10.1155/2012/127362. PMC 3368374. PMID 22690213.
Wakabayashi H, Sakuma K (May 2014). “Comprehensive approach to sarcopenia treatment“. Current Clinical Pharmacology. 9 (2): 171–80. doi:10.2174/1574884708666131111192845. PMID 24219006.
Finkle WD, Greenland S, Ridgeway GK, Adams JL, Frasco MA, Cook MB, et al. (29 January 2014). “Increased risk of non-fatal myocardial infarction following testosterone therapy prescription in men“. PLOS ONE. 9 (1): e85805. Bibcode:2014PLoSO...985805F. doi:10.1371/journal.pone.0085805. PMC 3905977. PMID 24489673.
Vigen R, O‘Donnell CI, Barón AE, Grunwald GK, Maddox TM, Bradley SM, et al. (November 2013). “Association of testosterone therapy with mortality, myocardial infarction, and stroke in men with low testosterone levels“. JAMA. 310 (17): 1829–36. doi:10.1001/jama.2013.280386. PMID 24193080.
Basaria S, Coviello AD, Travison TG, Storer TW, Farwell WR, Jette AM, et al. (July 2010). “Adverse events associated with testosterone administration“. The New England Journal of Medicine. 363 (2): 109–22. doi:10.1056/NEJMoa1000485. PMC 3440621. PMID 20592293.
Robinson SM, Reginster JY, Rizzoli R, Shaw SC, Kanis JA, Bautmans I, et al. (August 2018). “Does nutrition play a role in the prevention and management of sarcopenia?“. Clinical Nutrition. 37 (4): 1121–1132. doi:10.1016/j.clnu.2017.08.016. PMC 5796643. PMID 28927897.
Bauer J, Biolo G, Cederholm T, Cesari M, Cruz-Jentoft AJ, Morley JE, et al. (August 2013). “Evidence-based recommendations for optimal dietary protein intake in older people: a position paper from the PROT-AGE Study Group“. Journal of the American Medical Directors Association. 14 (8): 542–59. doi:10.1016/j.jamda.2013.05.021. PMID 23867520.
Deutz NE, Bauer JM, Barazzoni R, Biolo G, Boirie Y, Bosy-Westphal A, et al. (December 2014). “Protein intake and exercise for optimal muscle function with aging: recommendations from the ESPEN Expert Group“. Clinical Nutrition. 33 (6): 929–36. doi:10.1016/j.clnu.2014.04.007. PMC 4208946. PMID 24814383.
Tessier AJ, Chevalier S (August 2018). “An Update on Protein, Leucine, Omega-3 Fatty Acids, and Vitamin D in the Prevention and Treatment of Sarcopenia and Functional Decline“. Nutrients. 10 (8): 1099. doi:10.3390/nu10081099. PMC 6116139. PMID 30115829.
Fujita, Satoshi; Volpi, Elena (1 January 2006). “Amino Acids and Muscle Loss with Aging“. The Journal of Nutrition. 136 (1): 277S–280S. doi:10.1093/jn/136.1.277S. ISSN 0022-3166. PMC 3183816. PMID 16365098.
Brioche T, Pagano AF, Py G, Chopard A (August 2016). “Muscle wasting and aging: Experimental models, fatty infiltrations, and prevention“ (PDF). Molecular Aspects of Medicine. 50: 56–87. doi:10.1016/j.mam.2016.04.006. PMID 27106402. S2CID 29717535.
Wu H, Xia Y, Jiang J, Du H, Guo X, Liu X, et al. (September 2015). “Effect of beta-hydroxy-beta-methylbutyrate supplementation on muscle loss in older adults: a systematic review and meta-analysis“. Archives of Gerontology and Geriatrics. 61 (2): 168–75. doi:10.1016/j.archger.2015.06.020. PMID 26169182.
Holeček, Milan (August 2017). “Beta-hydroxy-beta-methylbutyrate supplementation and skeletal muscle in healthy and muscle-wasting conditions: HMB supplementation and muscle“. Journal of Cachexia, Sarcopenia and Muscle. 8 (4): 529–541. doi:10.1002/jcsm.12208. PMC 5566641. PMID 28493406.
Sayer AA, Robinson SM, Patel HP, Shavlakadze T, Cooper C, Grounds MD (March 2013). “New horizons in the pathogenesis, diagnosis and management of sarcopenia“. Age and Ageing. 42 (2): 145–50. doi:10.1093/ageing/afs191. PMC 3575121. PMID 23315797.
Lynch GS (November 2004). “Emerging drugs for sarcopenia: age-related muscle wasting“. Expert Opinion on Emerging Drugs. 9 (2): 345–61. doi:10.1517/14728214.9.2.345. PMID 15571490. S2CID 73056527.
进一步阅读Further reading
Fujita S, Volpi E (January 2006). “Amino acids and muscle loss with aging“. The Journal of Nutrition (Review). 136 (1 Suppl): 277S–80S. doi:10.1093/jn/136.1.277S. PMC 3183816. PMID 16365098.
Roubenoff R (December 2007). “Physical activity, inflammation, and muscle loss“. Nutrition Reviews. 65 (12 Pt 2): S208-12. doi:10.1111/j.1753-4887.2007.tb00364.x. PMID 18240550.
Sharlo K, Tyganov SA, Tomilovskaya E, Popov DV, Saveko AA, Shenkman BS. (December 2021) “Effects of Various Muscle Disuse States and Countermeasures on Muscle Molecular Signaling“. International Journal of Molecular Sciences. 23(1):468. PMID 35008893 PMC 8745071 doi:10.3390/ijms23010468
本文是陶可版权所有,未经授权请勿转载。本文仅供健康科普使用,不能做为诊断、治疗的依据,请谨慎参阅
评论